Frequently Asked Questions - FAQ |
|
-
1 General information
-
2 Measurement Methods
-
3 Physiology
-
4 Application
1 General information
1.1 What is O2C (oxygen to see)? |
 |
The O2C (oxygen to see) was developed by LEA Medizintechnik. It
is a combination of a laser Doppler flowmeter and a tissue spectrometer. With
this device it is possible to measure simultaneously in different measurement
depths blood flow, blood flow velocity, oxygen saturation and relative amount of
haemoglobin in the measurement tissue. The O2C version O2C-OF is the succession
of the OptoFlow. The O2C version O2C-ATS is the succession of the AbTisSpec.
1.2 What is the OptoFlow? |
 |
The device named OptoFlow was developed by LEA Medizintechnik. It
contains a laser doppler flowmeter which measures by a patented procedure
simultaneously in different measurement depths with only one probe. The OptoFlow
has been integrated into the O2C (oxygen to see) in the year 2002.
1.3 What is the AbTisSpec? |
 |
The name AbTisSpec is an acronym for absorption tissue
spectrophotometer. This device was developed by LEA Medizintechnik. It is an
advancement of the EMPHO in the measurement of the microvascular oxygen
saturation of haemoglobin in the tissue. Additionally the AbTisSpec measures the
relative amount of haemoglobin in the tissue. The spectrophotometry of the
AbTisSpec has been increased from the detection of visible light to the
detection of near infrared radiation. This technology was integrated to the
O2C(oxygen to see) in the year 2002.
1.4 What is the EMPHO? |
 |
The name EMPHO is an acronym for the Erlanger microlightguide
photometer. This device was developed by the Institute of physiology and
cardiology Erlangen in the late 80's. The EMPHO is a tissue spectrometer which
is illuminating the tissue and calculates the microvascular oxygen saturation of
haemoglobin by detection of the backscattered light. In the 90's the EMPHO has
been produced and distributed by the company Diehl (Nürnberg) and following by
the company Bodensee-Gerätewerk (Überlingen). In the late 90's the production
has been given up.
1.5 How does it compare to other methods? |
 |
Method |
Parameter |
Disadvantage |
O2C oxygen to see |
determines oxygen saturation,
amount of haemoglobin and blood flow at the venous part of the
capillaries. Information about the metabolism of tissue |
local Microcirculation |
|
Blood gas analysis:
SvO2 |
determines mixed venous oxygen
saturation of the whole body or of one organ, depending on the vein. Does
not determine local oxygen saturation in tissue. |
global |
Heterogeneity of organ perfusion
and influence of shunt blood. |
Pulseoximetry |
determines arterial oxygen
saturation, and is a measure for saturation of blood with oxygen in the
lung, therefore the lung function. |
global |
No information about local hypoxia
(as venous saturation is not determined) and locally delivered amount of
oxygen (as perfusion not determined). |
Ultrasound Doppler |
determines blood flow in big
vessels. |
local Macrocirculation |
local heterogeneity in supplied
organ, microangiopathies; is only indirect indicator for hypoxia. |
Angiography |
determines anatomy of supply
vessels. |
local Macrocirculation |
hemodynamic relevance of stenosis,
local heterogeneity of organ; is only indirect indicator for
hypoxia. |
Plethysmography |
determines blood flow in whole
extremities |
local Macrocirculation |
|
Nail fold microscopy |
makes capillaries visible and
their pathologic changes. With additional video systems blood flow
velocity can be determined. |
local Microcirculation |
expensive, cannot be used
everywhere in the body, only determines flow in single capillaries. |
pO2 tissue oxygen partial
pressure |
determines pO2 transcutaneously or
with needle probes subcutaneously. Gives the mixed value of arterial,
venous and tissue-pO2. |
local Microcirculation |
transcutane electrode influences
measurement by heating, needle probe by tissue trauma. Both are influenced
easily by arterial pO2, so that hypoxia in critical venous areas ("lethal
corner") can be overseen. Reason for pO2 change (delivery or consumption)
cannot be determined. |
NIR-Spectrometry |
determines optically oxygen
saturation, especially in capillary-venous area of microcirculation
similar to the spectrometric method that is used by the O2C. |
local Microcirculation |
Reason for SO2 change (delivery or
consumption) cannot be determined. |
Laser Doppler |
determines blood flow in the
microcirculation either punctual or by a scanner over a certain tissue
area. |
local Microcirculation |
low penetration depth,
instability. Indirect indicator for hypoxia. |
1.6 Is it reproducible? |
 |
Several studies concerning reproducibility, evaluation and
validation had been conducted for the O2C (oxygen to see).
Among others the
correlation with Microspheres and cerebral venous oxygen saturation had been
shown. Blood flow velocity, as well as local oxygen saturation measured with O2C
correlated well with perfusion measurement by microspheres and cerebral venous
oxygen saturation.
Reproducibility Studies had shown no significant changes
during this observation period.
2 Measurement Methods
2.1 Why does O2C not show 100% oxygen saturation? What
is the difference to the pulse oximeter? |
 |
O2C measures in the capillary-venous part of the vascular tree.
These are the vessels that carry the blood back from the organ to the heart
after distribution of oxygen and nutrients to the cells. Therefore they carry
the oxygen which was not consumed by the cells. This oxygen reflects the "oxygen
reserve". In the whole body it is about 75%. Still in the microcirculation it
can be much lower, because of local heterogeneities. Undersupply ("hypoxia")
occurs first in the capillary-venous areas ("lethal corner"), therefore O2C is
very sensitive to tissue hypoxia.
Pulse oximetry determines arterial oxygen
saturation, that means the amount of oxygen that is in the vessels leading to
the organs. Arteries transport oxygen from the lung (through the heart) to the
tissue, before distribution of the oxygen to the cells. Therefore arterial
oxygen saturation is a measure for oxygen saturation of blood in the lung, and
therefore lung function. Arterial oxygen saturation is about 98-100% in a person
with healthy lungs. With pulse oximeter local oxygen undersupply cannot be
determined, as hypoxia occurs after distribution of oxygen to the organs in
areas with the lowest oxygen saturation, the capillary-venous area.
The
difference between arterial and venous oxygen saturation ("arterial-venous
difference"), that means the oxygen extraction, amounts to 25% in the whole
body. This applies for the macrocirculation, if blood is drawn from aorta and
caval vein. The venous blood includes "shunt-blood" (blood that has not passed
oxygen consuming cells, but is directly flowing from arteries into veins) and
blood of organs with completely different oxygen extraction (high heterogeneity
of organs). Therefore it does not allow determination of oxygen extraction of
certain organs.
To determine extraction of single organs it would be
necessary to draw blood from the artery and vein of the organ of interest. The
difference would be the local oxygen extraction. Still it is often not feasible
to draw blood of single organs. Also in the organ there are also heterogeneities
that are not reflected by oxygen measurements in single vessels. With O2C,
however, it is possible to determine capillary-venous oxygen saturation and
therefore extraction, if arterial oxygen saturation is known. Now it is possible
to determine changes in local oxygen extraction and together with blood flow
oxygen consumption.
For determination of changes of local oxygen consumption
(metabolism), it is necessary to measure both, local oxygen saturation and local
blood flow.
2.2 What is the difference to the pO2
Electrode? |
 |
The pO2 is a common measure for dissolved oxygen in tissue. It
depends on constant parameters like solubility coefficient of blood and cells,
and also on the amount of oxygen that is released by haemoglobin, the oxygen
carrier in blood. Under normal conditions almost all oxygen is carried by
haemoglobin to the tissue and the capacity of blood to carry oxygen physically
dissolved is very low. In the tissue the oxygen is released by the haemoglobin,
physically dissolved and diffuses into tissue. If haemoglobin is highly
saturated with oxygen a small drop in oxygen saturation causes a big drop in pO2
according the "oxygen binding curve". Therefore changes in pO2 do not reflect
actual changes in oxygen saturation and amount of released oxygen. The amount of
oxygen that is transported into tissue therefore is only characterised by
changes in oxygen saturation.
Oxygen saturation usually drops in a linear
way along the organ supplying vessels (as each unit of tissue consumes the same
amount of oxygen), whereas pO2 drops exponentially (due to the hyperbolic oxygen
binding curve).
pO2 electrodes determine a mixed value of arterial, venous
and tissue pO2. Because of the exponential drop, the influence of the arterial
part of the vessel system is higher than changes in tissue or capillary pO2.
Hypoxic areas can be overseen.
As pO2-electrodes determine present amount of
oxygen, they do not distinguish between oxygen supply and
consumption.
Transcutaneous pO2 electrodes influence measurement results also
by heating of measurement area. Heating causes vasodilatation and increasement
of blood flow. Therefore oxygen extraction decreases and values shift to more
arterial oxygen saturation. Subcutaneous pO2 electrodes influence measurements
by insertion into tissue and cause tissue trauma.
2.3 What is the difference between tissue spectroscopy
with two wavelengths and oxygen measurement of O2C with several hundreds
of wavelengths? |
 |
A similar method like oxygen saturation measurements with O2C is
near-infrared spectroscopy as used by NIRO of Hamamatsu. An important difference
to the O2C is the measured and processed wavelength range.
For that you have
to know something about influence of tissue on light passing through tissue. On
its way through tissue light is absorbed by different tissue chromophores and
partly scattered by mitochondria. As well absorption as scattering changes the
amount of light of certain wavelengths, that is returning from tissue to the
detector and can be measured. Absorption, that is mainly caused by blood, causes
certain changes in spectra of the whole measured wavelength range, as well as
scattering and other tissue chromophores cause specific changes.
Certain
wavelengths are more influenced by one or another factor. If you are only
looking at a few wavelengths it is difficult to determine which factor caused
the change in intensity of measured light and different properties of light have
to be used for calculation (e.g. phase differences of light). But if you observe
the whole wavelength range, you can quantify the influence of different factors
by analysis of shape changes of the spectra. O2C determines all wavelength of
visible range that are changed by blood. Therefore changes in scattering
properties of the tissue, that influence spectra at specific wave lengths, can
be determined in each measurement and taken into account in each calculation.
2.4 What is measurement depth of O2C? |
 |
This questions can only be answered at the moment by mathematical
calculations and measurements at models. Light moves in tissue more easily in
the forward direction than in the backward direction. That means that light that
is immitted into tissue e.g. a finger rather can be seen at the other side of
the finger coming out, than at the side where the light has been immitted. This
makes measurements in "remission" - back to the surface - more difficult than in
"transmission" through tissue. Light that is backscattered to the surface moved
- much simplified - in a semicircular path through tissue. Therefore measurement
depth mainly depends on the distance of immitting to detecting lightguide. In
near-infrared wavelength range mathematical models showed, that a glass fibre
separation of 400-800 µm collects light of blood of the upper dermis (1). With a
separation of 2,5 cm a measurement depth of 2 cm in brain could be achieved (2).
In a model with Intralipid (2%) and haemoglobin (0.28 g/dl) a measurement depth
of 3.4 mm with a separation of 6 mm could be shown (3). Measurement depth
depends on optical properties of tissue, of amount of blood and probe geometry.
The exact measurement depth therefore cannot be determined, but it is clear,
that measurements with two different separations allow two different measurement
depths (deep and superficial), as possible with the O2C probes. That is the way
it should be described. Physiological reactions (e.g. perfusion increase during
work) indicate, that with current design muscle perfusion can be determined with
deep measurement depths. The difference to laser Doppler scanning has to be
emphasised: laser Doppler scanning is a method that produces maps of perfusion.
As here light is not directly coupled into tissue, mainly the part of the light
is measured, that is reflected at the surface of skin and has a penetration
depth of few micrometers. Measurement volume is accordingly small and
unrepresentative, a problem that can only in part be compensated by scanning
technique.
- 1 Meglinksky IV, Matcher SJ. Modelling the sampling volume for skin blood
oxygenation measurements
Med Biol Eng Comput, 39(1):44-50, 2001
- 2 Luo Q, Nioka S, Chance B. Functional Near-Infra Red Imager
SPIE 2979:
84- 93, 1997
- 3 Dissertation, Alfons Krug, Quantitative optische Gewebemessungen am
Herzen und an der Leber
Friedrich-Alexander-Universität Erlangen-Nürnberg,
p. 73-84. 1998
2.5 How fast is O2C? |
 |
Update of measurement values in monitoring window each two
seconds.
Update in beat-to-beat window each 50 ms.
For comparison:
Conventional pO2-Electrode: about 20 minutes equilibration time.
3 Physiology
3.1 How does O2C measure capillary-venous and what
information does that provide? |
 |
Information of the capillary venous area of the vessels, meaning
the vessels that transport blood after distribution of oxygen to the
organs/cells, is gathered with the O2C. The reason is, that light is absorbed by
vessels bigger than about 100 µm and does not return to the detector system (1).
Therefore only the smallest vessel of nutritive organ supply (microcirculation)
is measured (arterioles, capillaries, venoles). As about 85% of the blood is in
the capillary-venous system (2), O2C measurements represent mainly this area.
Therefore oxygen supply is determined locally in the organ and not globally.
- 1 Gandjbakhche, A.H., Bonner, R. F., Arai, A. E., Balaban, R. S.,
Visible-light photon migration through myocardium in vivo
Am. J. Physiol.
277 (46): H698-H704, 1999
- 2 Burton A.C., The vascular bed. In: Physiology and biophysics of the
circulation
Chicago, IL: Year Book Medical, 1965
3.2 Why is it important to measure simultaneously
oxygen saturation and blood flow? |
 |
The measured oxygen saturation is the percentage amount of oxygen
bound on haemoglobin.
This is important for the diagnosis of tissue hypoxia,
as the physically dissolved oxygen can be measured directly by a measurement of
oxygen saturation. This is made possible by the oxygen binding curve of the
haemoglobin.
If you want to know, how much oxygen is absolutely available,
you additionally need the blood flow. With this information it is possible to
determine oxygen uptake by tissue, when absolute amount of inflow (by blood flow
and arterial oxygen saturation) and absolute amount of outflow (by blood flow
and capillary venous oxygen saturation) can be determined.
Reasons for
decreased oxygen saturation, that are increased oxygen uptake or decreased blood
flow, can be determined.
If you measure blood flow only, you can determine
the amount of delivered oxygen. But there is no information gained about
availability of oxygen in the capillary venous system.
4 Application
4.1 Can the probe be used intracorporally? |
 |
The probes can be used in the gastrointestinal tract with an
endoscope, a feeding tube, a plastic protective sleeve or without any aid.
4.2 What is important for application of the
probe? |
 |
Blood flow measurements are influenced by movement. Application
without any movement is necessary for valid measurements. SO2 and Hb
measurements are independent of movement and therefore stable even under
difficult conditions. Frequent mistakes during blood flow measurements are:
- Movement at the probe tubing due to insufficient strain relief
- Movement artefacts by respiration movements, if the hand or arm is lying
on thorax or touching it (particularly difficult during measurements in the
gut or moving organs)
- Not visible muscle shivering of the patient.
Oxygen saturation measurements are influenced by colours and
light:
- Frequent mistakes: coloured disinfectant (there is also uncoloured
disinfectant in the OR, if you insist on it), especially directly after
surgery at intensive care units (wash of the colour before measurement).
- Influence of external light: Cover probe with blanket, if direct surgery
light shines on measurement point. Always control hemoglobin spectra (correct
spectra see manual)!!
All measurements of microcirculation are influenced by
pressure:
- Frequent mistake: Application of the probe with too much pressure on
tissue. Radial application of tape can cause venous congestion!
4.3 What do my measurements mean? |
 |
Provocation, therapy or disease leads to:
Symptom |
Blood flow |
Haemoglobin
amount |
Oxygen
saturation |
Cause |
1. Slow decrease of blood flow |
Slow decrease of blood flow |
Increase of haemoglobin amount |
Slow decrease of SO2 |
-> Venous congestion |
2. Decrease of blood flow and
SO2 |
Decrease of blood flow |
Small decrease or no change of
haemoglobin amount |
Decrease of SO2 |
-> Arterial ischemia |
3. Measurement in a tumour: |
High blood flow |
High haemoglobin amount |
Low SO2 |
-> high metabolism |
4. Measurement in an open
wound: |
High blood flow |
High haemoglobin amount |
High SO2 |
-> hyperaemia as sign of
inflammation |
5. A diabetic patient shows
following parameter at the leg: |
High blood flow |
Normal (or high) haemoglobin
amount |
High SO2 |
-> Hyperaemia caused by
vegetative neuropathy |
6. An arteriosclerotic patient
shows following parameter at the leg: |
Low blood flow |
Low haemoglobin amount |
Low SO2 |
-> arterial ischemia |
7. An arteriosclerotic patient
shows following parameter at the leg: |
Low blood flow |
Low haemoglobin amount |
Very high SO2 |
-> Tissue with low metabolism
caused by ischemic damage |
8. An arteriosclerotic patient
shows following parameter at the leg: |
no stable values and a spectrum as shown here:
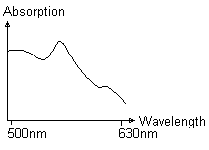 |
The spectrum is no haemoglobin
spectrum, but a cytochrome spectrum of reduced cytochromes, i.e. the
tissue is no longer supplied by oxygen. The calculated values are
invalid! |
4.4 What are normal values? |
 |
Measurement values depend on skin temperature and emotional
activation of the patient. Following tables are showing reference values:
Finger/Toe:
|
Normal values |
Critical values |
rHb |
35-90 AU |
<15 AU oder >90 AU |
SO2 |
70-90% |
<10% |
Blood Flow |
10-200 |
<5 AU |
Arm/Leg:
|
Normal values |
Critical values |
rHb |
35-90 AU |
<15 AU oder >90 AU |
SO2 |
20-50% |
<10% |
Blood Flow |
10-50 |
<5 AU |
Perfusion at different skin sites in comparison:
Finger(warm)
> Face > Arm > Leg
4.5 What does AU mean? |
 |
The abbreviation A.U. used for the units of blood flow and blood
flow velocity means "Arbitrary Units". These are units that are chosen
arbitrarily by the developer of the device.
The reason for the introduction
of "Arbitrary Units" is based on the origin of the values. The measured signals
for blood flow are electrical values of frequencies and amplitudes, so that the
unit would be a combination of electrical units. Therefore usually a new unit
for blood flow is introduced. To calculate the blood flow in ml/min, it would be
necessary to compare the electrical signals with a method that measures the
blood flow in ml/min (e.g. plethysmography, microspheres) for each organ (or
organs with similar optical properties). Then the arbitrary units can be
converted in ml/min. This "calibration" has to be done at the measured organ, as
there is no artificial model at the moment, that simulates tissue in a realistic
way.
The same applies for the unit of haemoglobin rHb [A.U.].
4.6 Does ambient light affect the readings? |
 |
No, normally the probe darkens an area big enough, so ambient
light does not affect the readings. The O2C (oxygen to see) displays the
haemoglobin spectra online at the screen. So you can always check whether you
got an adverse effect by ambient light.
The laser-Doppler measurements of the
O2C (oxygen to see) has an ambient light compensation algorithm. Nevertheless
the measurement can be influenced by ambient light.
4.7 What resolution does the measurement have? |
 |
Oxygen saturation
Measurement range 0- 100 % absolute
measurement
Resolution +/- 1 %
Amount of
haemoglobin
Measurement range 0 - 120 AU (arbitrary unit) relative
measurement
Resolution +/- 1 AU
blood flow
velocity
Measurement range 0 - 4000 AU (arbitrary unit) relative
measurement
Resolution +/- 1 AU